|
|
Genetic Analysis of Variation in Neuron Number Richelle Cutler
Strom
Chapter 4: Genetic and
Environmental Control of Retinal Ganglion Cell Variation
Introduction
Evidence of global genetic control over cell number is found from the
linear scaling of brain structures with brain size across species (Finlay
and Darlington, 1995). There is also evidence of localized genetic control
over cell number within particular brain structures. The genetic control
within brain structures is necesssary for the conservation of neuron number
in the event of species-specific adaptations. Some examples of
species-specific adaptations associated with neuron number are the capacity
for bird song and foraging memory in birds, and the lateralization of
language in humans. With each species adaptation, selective pressures for
behavior must have acted upon the natural variation in the neuron population
within the species. Examining the genetic bases of variation in
discrete cell population within a species will shed light on the genetics of
species-specific adaptations in neuron number. In this study, I examine the
genetic and environmental control of variation in a single well-defined
neuron population, the retinal ganglion cell population, among different
inbred strains of mice.
Retinal ganglion cells are the sole projection neurons relaying visual
information from the eye to the brain via their axons in the optic nerve.
The morphological and physiological attributes of retinal ganglion cells
have been described in many different species (Rodieck and Brening, 1983).
The relative simplicity of the retinal circuitry and its accessibility make
the retina an attractive model system for studying CNS development.
Consequently, a lot is known about the development of the retina, and in
particular ganglion cell differentiation. The differentiation of a large
portion of ganglion cell has been shown to require a POU-domain
transcription factor, Brn-3b (Gan, 1996). Other factors that may be
involved in ganglion cell differentiation are fibroblast growth factor
(Guillemot and Cepko, 1992) and the transcription factor RPF-1 (Zhou et al.,
1996).
Estimating numbers of distinct neuron populations can be tedious and
error prone. However, retinal ganglion cell number can be estimated
precisely and quickly by estimating the number of axons in the optic nerve.
This is important because the precision of heritability estimates and gene
mapping rely on the ability to obtain accurate phenotype data. In order to
map genes that control ganglion cell number, specifically, the variance
attributed to cofactors, such as brain weight, body weight, sex, and age,
needs to be minimized. In this chapter, I estimate the variance in ganglion
cell number associated with cofactors using linear regression. I also
examine the precision with which ganglion cell numbers can be estimated.
Finally, as a prelude to mapping QTLs responsible for variation in ganglion
cell number between mouse strains I determine the heritability of ganglion
cell number and the minimum number of effective genes.
Materials and methods
Mice
Optic nerves and brains were taken from mice, belonging to18 standard
inbred strains. All of these strains were obtained from the Jackson
Laboratory (Bar Harbor, ME). Optic nerves and brains were taken from 26 BXD
recombinant inbred strains and two groups of intercross progeny generated
from crosses between BALB/cJ and CAST/Ei (CCASF2)
and between BXD32 and CAST/Ei (32CASF2). I also
obtained optic nerves and brains from five isogenic F1
hybrids, (32CASTF1, CCASF1,
CAF1/J, B6AxCF1/J, PLSJF1).
The hybrids CAF1/J and PLSJF1
were obtained from the Jackson Laboratory and the remaining were generated
in the University of Tennessee animal colony. Data for the F1
hybrids and their parents can be found in the Table 4.3. In three of the
five hybrids, the parents have large differences in ganglion cell number.
For example, 32CASF1 progeny were generated from
parents BXD32 and CAST/Ei. The BXD32 strain has the highest ganglion cell
number measured among the strains (75,800 ± 1,980) and CAST/Ei has the
lowest ganglion cell number (45,000 ± 1,000).
Some of the strains studied carried mutations affecting the retina. Six
strains carried a mutation in the ß -phosphodiesterase gene (C3H/HeJ, C3H/HeSnJ,
CD-1, PL/J, SJL/J, and MOLD/Rk). A mutation in the ß -phosphodiesterase gene
leads to retinal degeneration (rd). Retinal degeneration in the most
extreme cases results in the complete loss of rod photoreceptors by 2 months
of age. However, it is not evident that rd affects ganglion cell
number, since all the strains carrying rd, with the exception of
MOLD/Rk, have average ganglion numbers and one strain even has a high
ganglion cell number. The following strains carry the albino allele of the
gene tyrosinase (A/J, AKR/J, BALB/cJ, CD-1, 129/J, 129/SvJ, NZW/LacJ, SJL/J).
Albino mice typically have a reduction in the proportion of retinal ganglion
cells with uncrossed projections, but there is no association between the
albino allele and adult ganglion cellnumber
(Rice et al., 1995).
Tissue Fixation
Optic nerves were dissected from mice transcardially perfused with EM
grade fixative. The optic nerves were processed in 96 well microtiter
plates. The procedure involved gradually dehydrating the nerves with
increasing concentrations of alcohol, staining with 2% osmium, and
infiltration with Spurr’s resin. Embedded nerves were thin-sectioned, placed
on formvar-coated grids, and stained with lead citrate and uranyl acetate.
Estimating ganglion cell number
Ganglion cell number was estimated by counting their axons within the
optic nerve. Previous studies have demonstrated that axon counts are
reliable estimates of ganglion cell number, (Chalupa et al., 1984; Lia et
al., 1986; Perry et al., 1983; Rice et al., 1995; Williams et al., 1986),
see table 2). Nerves were photographed in a grid pattern on a JEOL EX2000II
electron microscope typically at a magnification of ~
x12,000. The nerve area
was determined by photographing the entire nerve at low magnification,
usually at 200x
or 250x.
Immediately after sampling the nerve at high magnification and photographing
the entire nerve at low magnification, the high and low magnifications were
calibrated by photographing a grid replica (2160 lines/mm, obtained from
EMS, Fort Washington, PA). Axons were counted within a 63 x 86 mm counting
frame drawn on the micrograph. To give unbiased estimates only those axons
intersecting the top and right edge of the frame were included in the count.
Total axon estimates were calculated by multiplying the mean axon density
(per micrograph area) by the total optic nerve area.
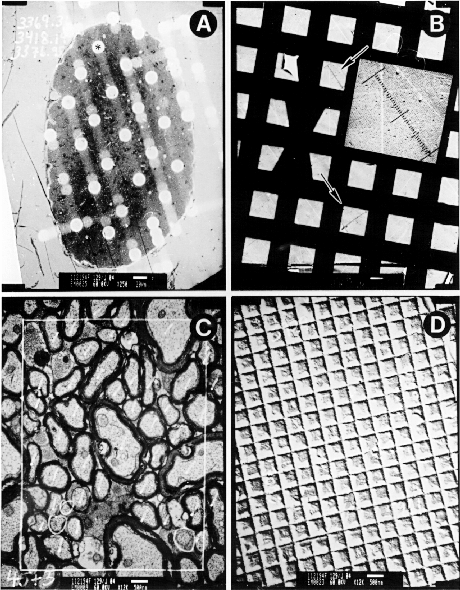
Figure 4.1. Method for estimating ganglion cell numbers. Ganglion
cell number for strains, species, and subspecies of mice. The major
subdivisions of mice are indicated in the left margin and are described in
the Materials and Methods. Numbers for each strain denote average ganglion
cell number, standard error of the mean (SEM) and the number of cases. These
four prints were made from negatives used to estimate ganglion cell number.
A and B are low-power electron micrographs of an optic nerve cross-section
(A) and calibration grid (B). C and D high-power electron micrographs of an
optic nerve sample site (C) and calibration grid (D). The white spots on the
nerve in A are areas that were bleached by the electron beam during sampling
at high-power. The asterisk in A marks the site that is shown at high
magnification in C. The axon margin was outlined with a sharpie marker using
the aid of a light microscope. The area of the nerve was calculated by
tracing the outlined nerve on a digitizing tablet. The two arrows in B mark
the sites where delimits spanning 80 grid units was etched into the negative
with a small scalpel and this distance measured under a dissecting
microscope with a digital caliper. Axons were counted within a counting
frame drawn in C. The axon count in this sample is 45 myelinated and 3
unmyelinated fibers (circled). The area of the counting frame was calibrated
using calibration grating in D. The dimensions of the calibration grid units
are 0.463 x 0.463.
Heritability
The broad-sense heritability of ganglion cell number was calculated from
the ratio of the genetic variance (VG) over the total phenotypic
variance (VP) found in the outbred strains. VG was
calculated by subtracting the average variance in ganglion cell number,
found within the two outbred strains (CD-1 and CARL/ChGo), by the average
environmental variance found within the inbred strains. The environmental
variance was obtained by averaging the variance within 18 inbred strains and
6 F1 hybrids. The average variance served to
reduce the effects of variance deviation resulting from unique
genotype–environment interactions. A close approximation to heritability in
the narrow-sense was made using the Hegmann and Posidente (1981) method. The
number of effective loci influencing ganglion cell number in the F2
intercross mice was calculated using the Castle-Wright equation.
Results
Variation among strains, species and subspecies
The ganglion cell number among standard inbred strains of mice range from
50,600 in A/J to 69,000 in BXD32 (Table 4.1). The average ganglion cell
number for the 18 standard inbred strains is 59,300 ± 1130 (n = 150).
The average coefficient of variation (CV) for inbred
strains is 6.9%. The variance among strains is much greater than within
strains (F(16,119) =15.0, p < 0.0001).
There are large differences in ganglion cell number between closely
related inbred strains. For example, the strains 129/J and LP/J originated
from a common ancestor in the mid-1920s, but their average ganglion cell
population differs by ~17,000. A difference of 11,000 ganglion cells was
discovered between shipments of C57BL/6J mice from the Jackson Laboratory.
The different shipments of C57BL/6J originated from different breeding rooms
at the Jackson Laboratory. No non-genetic factors were identified that could
explain the differences in ganglion cell number of the mice residing in the
two rooms. Thus, the difference in ganglion cell number is possibly due to a
new genetic mutation arising in the separate breeding colony.
Table 4.1. Average ganglion cell number for 18
standard inbred strains
Strain |
RGC |
SE |
SD |
CV |
n |
129/J |
63,772 |
±1,771 |
±4,339 |
6.8% |
7 |
129/SvJ* |
69,094 |
±5,391 |
±7,625 |
11.0% |
3 |
A/J |
50,615 |
±1,319 |
±3,490 |
6.9% |
8 |
AKR/J |
62,788 |
±935 |
±2,091 |
3.3% |
6 |
BALB/cBy |
55,859 |
±1,178 |
±3,331 |
6.0% |
9 |
BALB/cJ |
63,393 |
±2,290 |
±6,058 |
9.6% |
8 |
C3H/HeJ |
66,262 |
±3,857 |
±5,455 |
8.2% |
3 |
C3H/HeSnJ |
67,796 |
±1,448 |
±2,048 |
3.0% |
3 |
C57BL/6JAx1 |
66,082 |
±1,656 |
±4,684 |
7.1% |
9 |
C57BL/6J* |
55,413 |
±814 |
±4,071 |
7.3% |
26 |
C57BLKS/J |
65,667 |
±1,886 |
±4,217 |
6.4% |
6 |
C57L/J |
53,174 |
±2,654 |
±5,935 |
11.1% |
6 |
CBA/CaJ |
56,028 |
±1,203 |
±2,691 |
4.8% |
6 |
CE/J |
63,593 |
±2,536 |
±5,072 |
8.0% |
5 |
DBA/2J |
63,351 |
±1,208 |
±4,186 |
6.6% |
13 |
LP/J |
52,225 |
±1,989 |
±5,262 |
10.1% |
8 |
NZB/BinJ |
61,063 |
±1,600 |
±3,579 |
5.9% |
6 |
NZW/LacJ |
63,711 |
±727 |
±1,259 |
2.0% |
4 |
PL/J |
55,976 |
±1,309 |
±3,462 |
6.2% |
8 |
SJL/J |
52,473 |
±1,770 |
±3,958 |
7.5% |
6 |
Average |
60,417 |
±1,877 |
±4,141 |
6.9% |
7.5 |
*Pooled data from pigmented and coisogenic albino mice. The statistics
SD, SE, and CV were corrected for bias because of small sample size ( Sokal
and Rohlf, 1981). Data for the strain 129/SvJ is new and is not published in
Williams et al. (1996).
Ganglion cell number among species and subspecies ranges from 45,000 to
64,300. The average CV for these strains is 7.4% (Table 4.2). Note that the
strain CARL/RpGo is outbred and as expected has a higher degree of
variation, CV = 14%.
Table 4.2. Average ganglion cell number for inbred
representatives of wild strains
Strain |
Species |
RGC |
SEM |
SD |
CV |
n |
CASA/Rk |
M. m. castaneus |
47,205 |
±1,380 |
±2,390 |
5.1% |
4 |
CAST/Ei |
M. m. castaneus |
45,047 |
±1,040 |
±3,451 |
7.7% |
12 |
CZECHII/Ei |
M. m. musculus |
59,207 |
±3,482 |
±8,530 |
14.4% |
7 |
MOLD/Rk* |
M.m. molossinus |
43,758 |
±4,476 |
±8,952 |
20.5% |
5 |
WSB/Ei |
M.m. domesticus |
57,380 |
±1,759 |
±4,655 |
8.1% |
8 |
PANCEVO/Ei |
M.m. spicilegus |
64,300 |
±1,383 |
±3,093 |
4.8% |
6 |
SPRET/Ei |
M.m. spretus |
59,049 |
±1,896 |
±4,240 |
7.2% |
6 |
CARL/ChGo** |
M. caroli |
51,263 |
±2,270 |
±7,177 |
14.0% |
11 |
Average |
|
53,401 |
±2,211 |
±5,311 |
10.2% |
7.4 |
|
*MOLD/Rk carries the rd allele and there is a high incidence of
necrotic axons in the optic nerve of older mice. Estimates from the two
youngest MOLD/Rk cases (49,200 and 47,200) are probably better
representatives. **CARL/ChGo is an outcrossed strain.
The ganglion cell probability distribution of the 57 inbred strains,
including 19 inbred laboratory strains and 38 recombinant inbred strains
(Fig 4.2). The probability distribution has three modes at 55,500, 63,500,
and 75,500 and indicates that there are three distinct phenotypes. The third
mode consists of the strains BXD5 and BXD32, which have exceptionally high
ganglion cell numbers that are 20% above their high parental strain—DBA/2J.
A Kolmogorov–Smirnov goodness-of-fit test confirms that the inbred strain
distribution is not normally distributed (D = 0.245, p < 0.01)
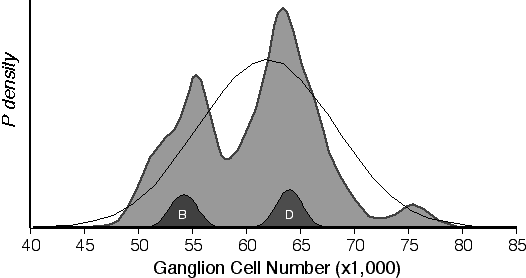
Figure 4.2. Probability density of retinal
ganglion cell number for 57 inbred strains.
The large filled-in function is a summation of individual normalized
probability densities of the ganglion cell averages from 57 strains. The two
small filled-in functions labeled B and D are examples of the individual
probability densities computed from C57BL/6J (B) and DBA/2J (D) strain
averages. The black line is the expected Guassian distribution based on the
mean of all strains (61,730 ± 6,310).
The variance in ganglion cell number within the heterogeneous crosses is
higher than the variance within the inbred strains (Table 4.3). The average
of corrected ganglion cell number in the CCAS F2
progeny is 56,000 ± 6000 (SD). The distribution of raw ganglion cell numbers
in the CCASF2 is broad, ranging from a low of
42,000 to a high of 70,000 (Fig 4.3). The broad range in ganglion cell
numbers indicates that multiple genetic factors have segregated that can
modify ganglion cell number. The number of genes modulating ganglion cell
number was estimated to be one in CCASF2 cross and
three in the 32CASF2 cross.
Table 4.3 Average ganglion cell population in
heterogenous mice.
Strain |
RGC |
SEM |
SD |
CV |
n |
CARL/RpGo |
51,263 |
±2,270 |
±7,177 |
14.0% |
11 |
CCASF2 |
56,501 |
±679 |
±7,156 |
12.7% |
112 |
32CASF2
|
62,020 |
±819 |
±9,656 |
15.6% |
140 |
*CD1/Go |
68,338 |
±2,183 |
±7,869 |
11.5% |
14 |
Average |
58,701 |
±1,710 |
±7,401 |
12.7% |
|
*CD1/Go is an outbred laboratory strain.
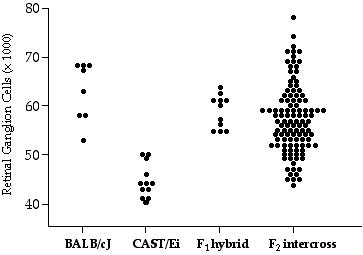
Figure 4.3. Scatterplot of retinal ganglion cell number for CCASF2,
CCASF1, and parental strains.
Technical variation
Reliability was estimated by sampling a set of 69 nerves two to four
times. Usually an adjacent nerve section was photographed and this was done
months to several years after the first count. The average of the first
count was 58,967 ± 1145, whereas that of the second count was 58,667 ±
1,361. The test-retest correlation coefficient was 0.83. The mean difference
between pairs of estimates was 4,080, and the average SD of sets of
replicates was 3,116. The technical coefficient of variation between
repeated samples of ~25 micrographs averaged 6.3 ± 0.5% SE. I calculated the
sampling variance across repeated samples and estimated that 60–70% of the
technical variance is associated with sampling error. Variance in
calibration errors and axon discrimination can account for the remaining
variance.
The coefficient of variation for the standard inbred strain averaged
6.9%. Thus, after taking into consideration the technical coefficient of
variation the corrected environmental coefficient of variation is 2.8%.
Correlation between neuron number, ages, sex, body weight, and brain
weight
The effects of age, sex, body weight and brain weight on ganglion cell
number within–strains were examined by calculating within strain z-scores
for retinal ganglion cell number and comparing this value to age, sex, body
and brain across strains. The correlation between age, sex, body weight,
brain weight and within–strain ganglion cell number within the 18 standard
inbred strains were approximately zero, (n = 124). In these strains,
the sex ratio was 50:50 and ages ranged from 30 to 200 days. The correlation
between brain weight and ganglion cell number across strains is +0.38, which
is highly significant, (t = 6.54, n = 184, p < 0.001). The
correlation between brain weight strain averages and ganglion cell strain
averages is +0.46, which is also significant at p < 0.02. The
corresponding coefficient of determination (r2)
is 21.5 %. The correlation between ganglion cell numbers and brain weights
regressed with respect to sex, age, and body weight is roughly the same,
r = 0.42.
Within BXD recombinant strains, ganglion cell number was not correlated
with brain weight, body weight, sex, or age. However, across strains
variation in ganglion cell number was significantly correlated with brain
weight, r = 0.42. The correlation coefficient was reduced to 0.36
when brain weight was corrected for variation in sex, age, and body weight.
In the CCASF2 cross variation in ganglion cell
number was significantly correlated with brain weight explaining 26.8% of
the variation in ganglion cell number (p = 0.0001). Body weight was
also significantly correlated with ganglion cell number (r2
= 11.5%, n = 92, p = 0.001), a product of the high correlation
between body and brain in CCASF2. However, adding
brain weight to the regression with body weight abolishes any correlation
with body weight. There was no correlation between ganglion cell number and
sex, age or parity in the CCASF2 cross. To reduce
the effect of brain weight variation on ganglion cell number, the ganglion
cell numbers of CCASF2 mice were standardized to
that of a 437 mg brain, the average brain weight of the F2
progeny. Correcting for differences in brain weight resulted in a 25%
reduction in variance of ganglion cell number among CCASF2
progeny. Variation in ganglion cell number was also significantly
associated with brain weight in the 32CASTF2
cross, but explained only 15.9% of the variation (p < 0.0001). There
was also no correlation between ganglion cell number and sex, age or parity
in the 32CASF2 cross.
Heritability and gene dominance
Broad-sense heritability calculated in outbred strains CD-1 and CARL/ChGo
is 0.73 and 0.68, respectively. Narrow-sense heritability of retinal
ganglion cell number in the standard inbred mouse strains is 0.48.
Narrow-sense heritability of ganglion cell number in the BXD strains is
0.47. Heritability for ganglion cell number in the CCASF2
cross is 0.90 and was reduced to 0.80 with corrected numbers. Heritability
of ganglion cell number in the 32CASTF2 cross is 0.50. Estimates
of the effective gene number modulating ganglion cell number are one in the
CCASF2 cross, 2.5 in the 32CASF2
cross, and 2.4 in the BXD strains. Due to the required assumptions for this
calculation, these estimates are likely to be underestimates of the true
gene number.
Three hybrids, CCASF1, 32CASF1,
and PLSJF1/J, exhibit dominance for high ganglion
cell number alleles (Table 4.4). For example, the average ganglion cell
number for CCAS F1 hybrids is 59,500 ± 3,400 (SD)
and is roughly 5,000 cells higher than the parental midpoint. The CV of
variation within the hybrids in Table 4.4 averages 5.7%. This CV average is
not significantly different from the CV of 6.9% found in the inbred strains
(one tailed t-test, p = 0.15). The heterozygous state in some
hybrids increases fitness that provides a buffer against developmental and
environmental effects. However, there is no evidence that the ganglion cell
number in these hybrids is more stable than in the homozygous strains.
Table 4.4. Average ganglion cell number in F1
hybrids and their parental strains
F1
Strains |
RGC ±
SE |
n |
Maternal strain |
RGC |
Paternal strain |
RGC |
Mid-Parental |
d |
CCASF1 |
59,457 ±
1117 |
32 |
BALB/cJ |
63,393 |
CAST/Ei |
45,047 |
54,439 |
5234 |
32CASF1 |
63,253 ±
916 |
7 |
BXD32 |
76,219 |
CAST/Ei |
45,047 |
60,633 |
2620 |
CAF1/J |
56,426 ±
1736 |
8 |
BALB/cJ |
63,393 |
A/J |
50,516 |
57,004 |
-578 |
B6AxCF1 |
63,731 ±
1944 |
18 |
C57BL/6Jax1 |
66,082 |
BALB/J |
63,393 |
64,738 |
-1007 |
PLSJF1/J |
56,096 ±
1333 |
25 |
PL/J |
55,976 |
SJL/J |
54,225 |
54,225 |
1871 |
Discussion
The significantly lower variance in ganglion cell number within strains
compared to across strains demonstrates that genetic differences are
involved in the variation in ganglion cell number among strains.
Heritability estimates for variation in ganglion cell number are high,
ranging from 0.80 in CCASF2 to 0.47 in the BXD
strains. Estimates of heritability within crosses generated from parental
strains that are widely divergent, such as BALB/cJ, and CAST/Ei parentals
for CCASF2, are typically inflated (Lynch and
Lynch, 1992). In addition, the estimates of broad sense heritability in the
intercrosses include the effects of gene dominance effects and epistatic
interactions among loci are in the neighborhood of 80%. Nevertheless, these
estimates are near the 80% estimate of genetic control over granule cell
number in the dentate gyrus of mice (Wimer and Wimer, 1989). Heritability
estimates such as these are based on ratios between genetic and nongenetic
variance. Consequently, minimizing environmental variance increases measured
heritability. In this study, all mice were reared in a pathogen-free
laboratory environmentÑa situation that eliminates numerous sources of
environmental differences, and that almost certainly increases estimates of
genetic control compared to dispersed wild populations of mice.
The ganglion cell numbers of CCASF1 and 32CASF1
exhibit dominance with respect to the high parental. This dominance could
result from hybrid vigor (see discussion in Chapter 2). In addition, the
dams that rear the F1 hybrids (BALB/cJ and BXD32)
have a larger body size compared to that of CAST/Ei. The BALB/J and BXD32
neonates are larger in size compared to CAST/Ei neonates. Case in point,
when reciprocal matings were performed, the CAST/Ei females were dieing in
labor trying to birth these larger size hybrid neonates untypical of their
species.The genetic factors producing a small body size in CAST/Ei could
have pleiotropic effects on brain weight and neuron numbers. Without the
body size restriction imposed, the CAST/Ei contribution the CCASF1
ganglion cell number might raise above the midparental range. The dominance
of the CCASF1 is also reflected in the
distribution of the CCASF2 (Fig. 4.3).
An analysis of isogenic animals makes it possible to assess the
consistency with which the genome guides the generation of traits such as
neuron number. When environmental differences are minimized, the residual
variance is due to microenvironmental effects and developmental noise. In a
systematic analysis of the grasshopper nervous system a remarkable level of
variation in neuron number was found both within and between isogenic groups
that had been reared in a tightly controlled environment. In one exceptional
group, half of the animals had the standard set of six ocellar interneurons
whereas the other half had seven to nine interneurons (Goodman, 1979). In
contrast, in a survey of optic nerves from a set of more than 100 isogenic
crustaceans (Macagno, 1980) found only a single exception to the rule of 176
axons. Thus, the developing nervous system is capable of of excruciating
control. However, it is not known how stringently large populations are
developmentally regulated in the vertebrate CNS. The average corrected
coefficient of variation in ganglion cell number is 2.8%. Thus, the
developmental control of ganglion cell number is stringent. Although,
coefficients of variation do range from 2.0% in NZW/LacJ to 11% in C57L/J
indicating that the degree of developmental stringency is under genetic
control. In addition, these differences could result from some of sampling
artifacts discussed in the methods
Variation in ganglion cell number was significantly correlated with brain
weight across strains and within the intercrosses. However, there was no
correlation between within strain z-scores and brain weight. The absense of
a correlation between ganglion cell number and brain weight within strains
suggests that the significant correlation found across strains is not due to
common non-genetic factors, such as the maternal environment. Thus, the
association of ganglion cell number and brain weight is due to the global
variation in cell number that originates early during development before the
divergence of specific cell lineages. Furthermore, the variation in brain
weight is a summation of the correlated variation of individual populations
as distinct as the retinal ganglion cells.
There was no decline in ganglion cell number with age in any of the mice
studied. This finding is corroborated by a study reporting no age-related
decline in ganglion number in the retina of young and old rhesus monkeys
(Kim et al., 1996). There are many reports of age-related decrease of
neurons in the cerebral cortex and cerebellum neuron populations, but there
are also many reports of no age-related decrease in neuron number within
distinct nuclei (Jacobson, 1991). In summary, a decline in neuron number is
not an inevitable result of aging and may depend on the endogenous level of
oxidative stress in a particular neuron type.
The bimodality of strain averages is a surprising and important finding
that provides evidence that there are single polymorphic genes that have
comparatively large effects on neuron number. This conclusion is
strengthened by the estimate from the Castle-Wright formula of one to three
genes with large effect size modulating ganglion cell in the F2intercrosses.
A speculation based on these findings is that variation in other neuron
populations may also be controlled by relatively small numbers of
quantitative trait loci that have major effects. The low gene number and
high heritability of ganglion cell number suggests that it should be
possible to map one or more genes with a quantitative effect on ganglion
cell number.
Return
to Table of Contents |
|
|